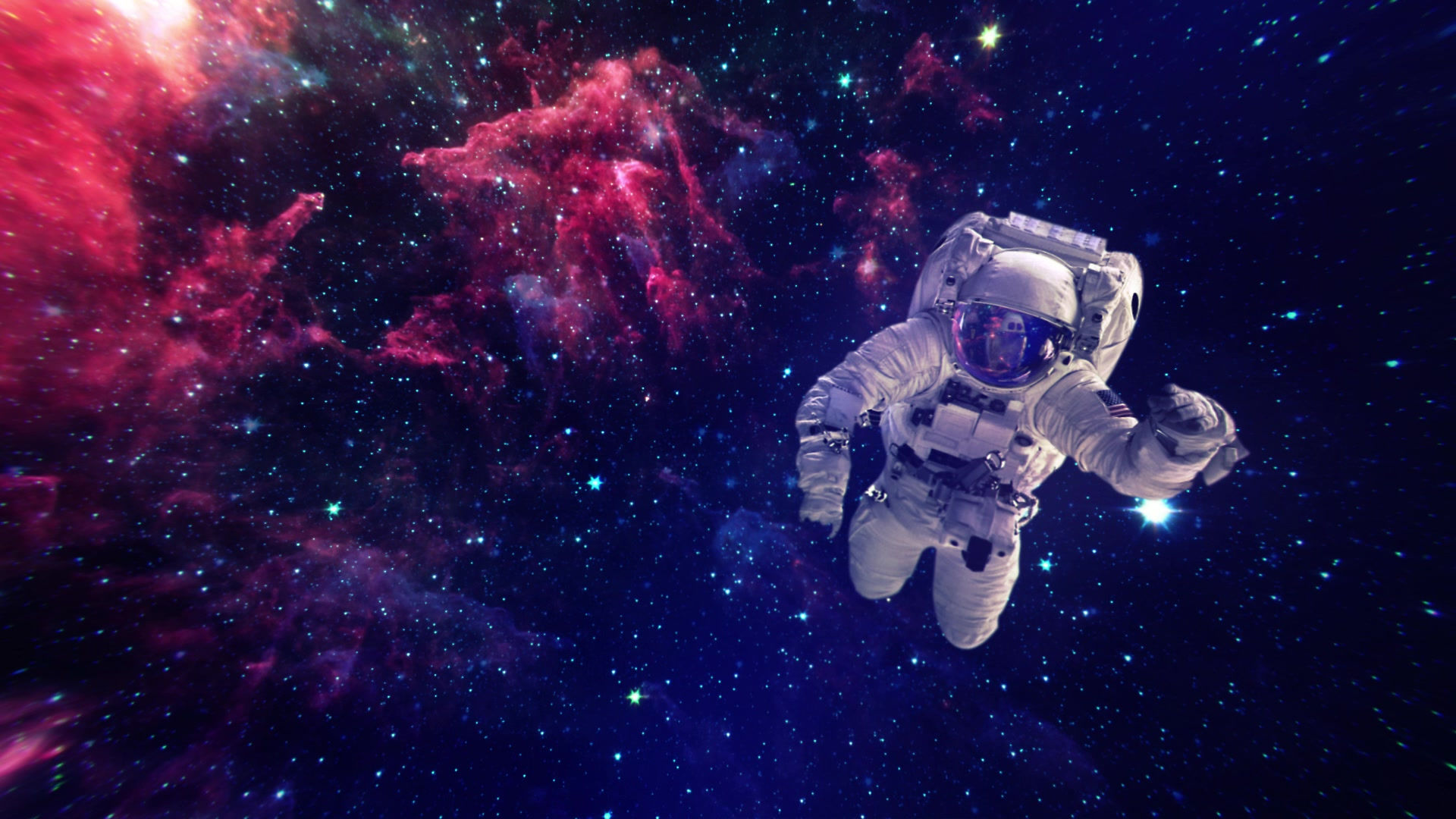
The Cost
The Value
The value of this system for all stakeholders can be characterized as progress. A bio-regenerative life support system will not only provide astronauts with a fresh diet that is sustainably grown, but it will also serve as a major step to long-term habitation in extreme environments and sustainable agriculture on Earth.
To us (the developers), the value of the project relies in its successful implementation. Because the system cannot be tested in the same operating conditions (specifically microgravity) due to the expense of space flight, the first long-duration mission on which the system is used will be the proof-of-concept. This first mission will be crucial because it will provide us with operational data. We will use this information to continuously work to improve the crop yield versus weight of the system in order to minimize cost and maximize the astronauts’ health and well-being in transit.
The direct user of the system would be the astronauts and the regulating space agency, in this case NASA. For the users, the value of the system comes in many forms. The value to the astronauts is their access to fresh, natural food. Replacing processed and rehydrated foods with fresh, nutrient-rich meals will increase the health of the astronauts. These fresh ingredients can be used to prepare any meal of the astronauts choice, increasing their mental health by making meals in space more enjoyable and representative of meals on Earth. Additionally, value from this project is realized in the system’s projected lifespan. Once the system is initialized, the only inputs are the occasional replenishment of nutrients and plants in the form of seeds. Assuming all waste and byproducts are recycled, the system can be considered self-sustainable. For NASA, this will be reflected in increased overhead costs/first costs for only one mission; thereafter, the system can be reused indefinitely for return missions, permanent colonies, etc.
Other stakeholders of this project will benefit from the implications that this technology has on the future of food production in space and on Earth. The testing phase of the project will verify the system’s usability and feasibility on Earth. The advancement and implementation of fogponics on Earth for either mass or personal food production would eliminate wastes and other components of modern agriculture that are harmful to the environment. Additionally, a successful proof-of-concept long duration mission will verify the usability of the system in any environment, including Mars and other colonization locations.
There is little room for value trade-offs for the system. The number of astronauts serves as a design point to the system. We were able to determine the optimal species of crops, the number of plants, and the corresponding size of the system to support the nutritional needs of four adult astronauts. The system can be scaled up to facilitate a larger number of astronauts (plants and size not necessarily linear, cost definitely not linear). However, trying to increase the size of the system for the same amount of astronauts in order to provide excess quantity and/or variety of food has been determined to be a detrimental trade-off. Because cost is exponentially dependent on weight, increasing the system size slightly will create a much larger cost increase.
​
​
There are several costs that need to be considered when determining the total cost of the system. These costs stem from all stages in project development and operation.
-
Operation (maintenance): The maintenance of this system includes the occasional monitoring the autonomous tending of the plants and the preparation of the crops into meals. Because the astronauts onboard the vehicle will take on these responsibilities, there is not monetary cost of maintenance. Rather, the cost of this maintenance will be in the form of the astronauts’ time.
-
Operation (support): Because any mission is heavily monitored 24/7 by ground crews, the new life support system is no exception. The cost of this support will include hiring a new support team in charge of monitoring the system and creating procedures for its use.
-
First costs: The first costs of the implementation of this system include the cost of development, system architecture and infrastructure, and the autonomous tending system. These costs will only occur once at the beginning of the product’s life.
-
Fuel: The added cost of fuel for the system is an exponential factor of the weight of system. Unlike first costs, the added cost of fuel recurs every launch.
The operation costs of the system would exceed that of the existing method of feeding astronauts. The current system does not have any direct operating or maintenance costs. However, the system’s operating costs would be less than that of an alternative solution, consisting of routine resupply missions to replenish the food supply. This solution is used for shorter duration transit missions or to colonized destinations. The operations of a single resupply mission would consist of a large ground support team, comparable to the operations of the bioregenerative system for the entire long-duration mission. Therefore the operating costs of periodic resupply missions would exceed the operating costs of the system. Due to the length of the mission to Mars, continued resupply missions may not be feasible, especially towards the latter end of the mission.
​
A manned mission to mars is estimated to cost $100 billion over the span of 30 to 40 years. At first glance, this may seem expensive. However, when compared with other spending, it seems far more viable. In 1969, during the height of the space race, NASA received 4.5% of the annual budget. Today, NASA receives just 0.5% of the federal budget. NASA received the same amount of funding as they did during the space race, they would have $159 billion per year to continue current programs and boost a mission to mars program. Additionally, the $100 billion required for this mission is small when compared to the annual military spending of $600 billion.
According to NASA, each astronaut consumes about 1.7 kg of food per day. Assuming a crew of four and a 30 month mission (6 month travel, 18-20 month for orbit realignment, 6 month return), about 6,120 kg of food would be the absolute minimum to get the crew to mars and back, given current propulsion methods are used. Using a bio regenerative system, this mass would be reduced. More importantly, this system would be able to continue producing food should there be a delay in the mission. Based on NASA’s current Veggie system, combined with our spaceponic system, we expect a 10 kg growing medium will house about 8 plants. This will be in addition to resources such as water, nutrients and tending structures. Altogether, the combined weight of the system is estimated to be under 2,000 kg. This would allow for significant weight reduction as well as discretion for NASA to utilize this as they please. Because the full 2,000 kg will not necessarily be utilized, this allows for some contingency mass to be added as deemed necessary.
Given the amount of research required to make this fogponic system the main food production source for long-term missions, much of the expected budget will go into research. This system cannot fail for any reason. In order to send about half a kg of food to the space station costs about $10,000. To launch 6,120 kg of food- on a mission to mars vehicle- into a transfer orbit would cost over $200 million, assuming it will be 50% more expensive to launch into this orbit. In order to complete all research, we have established a budget of $150 million. This would cost less than sending food with astronauts but also lead to new advancements in space-based agriculture. Providing more resources to the research, we could feasibly build a final product using less than $30 million. This would provide $120 million in funding for over ten years of research to ensure reliability.